Microfluidics and microfluidic devices
1. Whole-Teflon microfluidic chips (Published on PNAS)
Abstract
Although microfluidics has shown exciting potential, its broad applications are significantly limited by drawbacks of the materials used to make them. In this work, we present a convenient strategy for fabricating whole-Teflon microfluidic chips with integrated valves that show outstanding inertness to various chemicals and extreme resistance against all solvents. Compared with other microfluidic materials [e.g., poly(dimethylsiloxane) (PDMS)] the whole-Teflon chip has a few more advantages, such as no absorption of small molecules, little adsorption of biomolecules onto channel walls, and no leaching of residue molecules from the material bulk into the solution in the channel. Various biological cells have been cultured in the whole-Teflon channel. Adherent cells can attach to the channel bottom, spread, and proliferate well in the channels (with similar proliferation rate to the cells in PDMS channels with the same dimensions). The moderately good gas permeability of the Teflon materials makes it suitable to culture cells inside the microchannels for a long time.
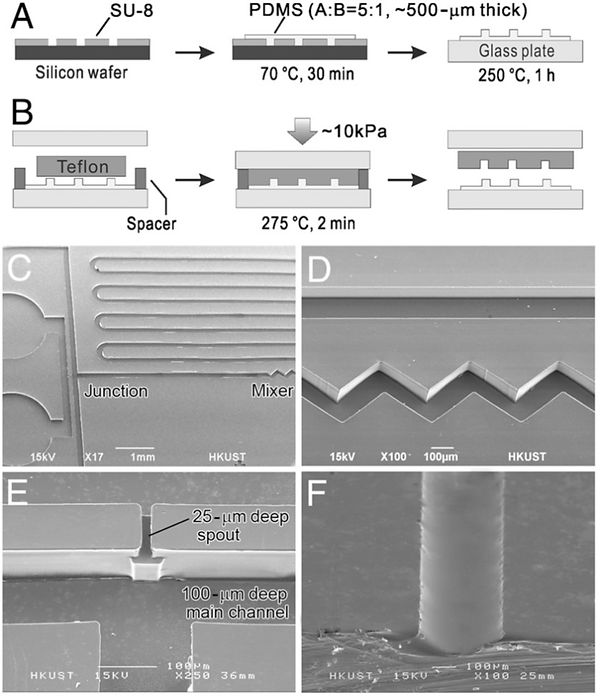
Molding of Teflon microchannels using PDMS masters. (A, B) Schematics
of the preparation of thermally stable PDMS masters and the molding
of Teflon channels, respectively. (C–E) SEM images of microfabricated PFA
channels (C) for droplet generation that includes a micromixer (D) and a
3-D spout (E). (F) Microchannel with a rounded profile molded from a reflowed
positive photoresist (AZ4903) structure.
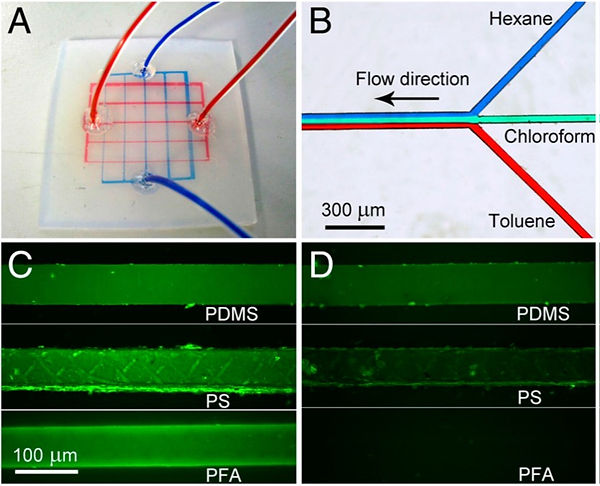
Solvent-resistance (A, B) and antifouling property (C, D) of Teflon channels. (A) A PFA chip with two-layer microchannels separated by a thin FEP membrane that are filled with acetone (colored with a red dye) and DMSO (colored in a blue dye). (B) Laminar flow of dyed organic solvents in a whole-Teflon chip. (C) Fluorescence images of different kinds of microchannels filled with a 100 μg∕mL GFP aqueous solution. (D) Fluorescence images of the channels in C after washing with buffer for 1 min.
2. Chemical Recognition in Cell-Imprinted Polymers (Published on ACS Nano)
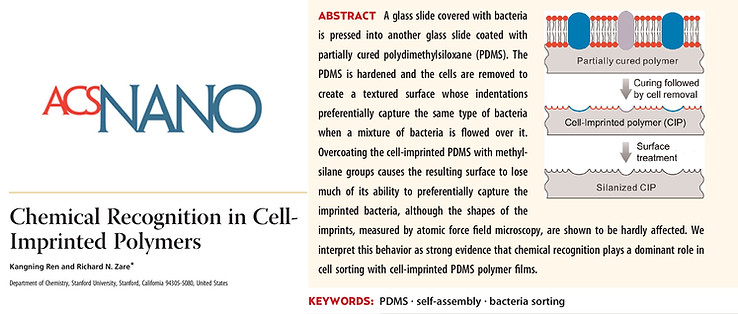
Abstract
A glass slide covered with bacteria is pressed into another glass slide coated with partially cured polydimethylsiloxane (PDMS). The PDMS is hardened and the cells are removed to create a textured surface whose indentations preferentially capture the same type of bacteria when a mixture of bacteria is flowed over it. Overcoating the cell-imprinted PDMS with methylsilane groups causes the resulting surface to lose much of its ability to preferentially capture the imprinted bacteria, although the shapes of the imprints, measured by atomic force field microscopy, are shown to be hardly affected. We interpret this behavior as strong evidence that chemical recognition plays a dominant role in cell sorting with cell-imprinted PDMS polymer films.
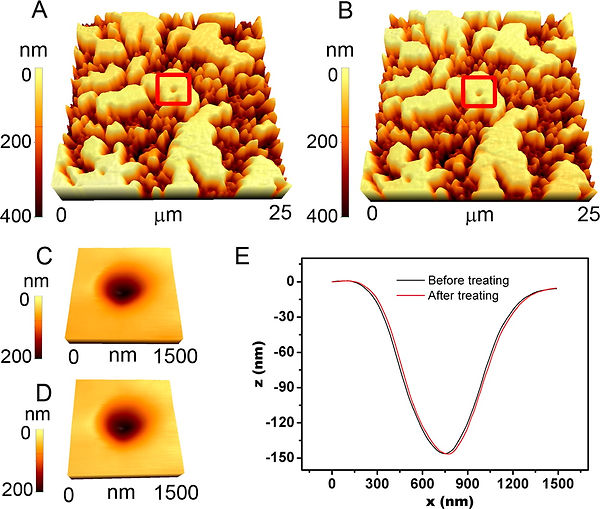
AFM images of a same location on the PDMS surface containing imprints of Staphylococcus aureus: (A) before silanization, and (B) after silanization. Close-ups of the areas marked with red squares in panels A and B are shown in panels C and D and the profiles of these areas are presented in panel E.
3. A one-step strategy for ultra-fast and low-cost mass production of plastic membrane microfluidic chips (Published on Lab on a Chip, 2016)

Abstract
An ultra-fast, extremely cost-effective, and environmentally friendly method was developed for fabricating flexible microfluidic chips with plastic membranes. With this method, we could fabricate plastic microfluidic chips rapidly (within 12 seconds per piece) at an extremely low cost (less than $0.02 per piece). We used a heated perfluoropolymer perfluoroalkoxy (often called Teflon PFA) solid stamp to press a pile of two pieces of plastic membranes, low density polyethylene (LDPE) and polyethylene terephthalate (PET) coated with an ethylene-vinyl acetate copolymer (EVA). During the short period of contact with the heated PFA stamp, the pressed area of the membranes permanently bonded, while the LDPE membrane spontaneously rose up at the area not pressed, forming microchannels automatically. These two regions were clearly distinguishable even at the micrometer scale so we were able to fabricate microchannels with widths down to 50 microns. This method combines the two steps in the conventional strategy for microchannel fabrication, generating microchannels and sealing channels, into a single step. The production is a green process without using any solvent or generating any waste. Also, the chips showed good resistance against the absorption of Rhodamine 6G, oligonucleotides, and green fluorescent protein (GFP). We demonstrated some typical microfluidic manipulations with the flexible plastic membrane chips, including droplet formation, on-chip capillary electrophoresis, and peristaltic pumping for quantitative injection of samples and reagents. In addition, we demonstrated convenient on-chip detection of lead ions in water samples by a peristaltic-pumping design, as an example of the application of the plastic membrane chips in a resource-limited environment. Due to the high speed and low cost of the fabrication process, this single-step method will facilitate the mass production of microfluidic chips and commercialization of microfluidic technologies.

Peristaltic-pumping operations. (A) and (B) are bright field images of the squeezing operation on a membrane chip. At the beginning of the introduction of crystal violent dye solution into the microfluidic system, some air bubbles were trapped inside the channels (arrows), especially in the corner of the sinuate channels. After squeezing the bubbles using a PDMS cylinder, the channels were fully filled with the solution (B). (C) Design of three microfluidic channels used for peristaltic-pumping operation tests with different volume-determining chambers. (D) The plot of the total volume of delivered ethanol solution versus the number of cycles of squeezing operation. Scale bars in A and B are 2 mm.
4. Cell-on-hydrogel platform made of agar and alginate for rapid, low-cost, multidimensional test of antimicrobial susceptibility (Published on Lab on a Chip, 2016)
Abstract
Antimicrobial resistance (AMR) is a rapidly increasing threat to the effective treatment of infectious diseases worldwide. The two major remedies include: (1) using narrow-spectrum antibiotics based on rapid diagnosis; and (2) developing new antibiotics. A key part of both remedies is the antimicrobial susceptibility test (AST). However, the current standard ASTs that monitor colony formation are costly and time-consuming and the new strategies proposed are not yet practical to be implemented. Herein, we report a strategy to fabricate whole-hydrogel microfluidic chips using alginate-doped agar. This agar-based microfabrication makes it possible to prepare inexpensive hydrogel devices, and allows a seamless link between microfluidics and conventional agar-based cell culture. Different from common microfluidic systems, in our system the cells are cultured on top of the device, similar to normal agar plate culture; on the other hand, the microfluidic channels inside the hydrogel allow precise generation of linear gradient of drugs, thus giving a better performance than the conventional disk diffusion method. Cells in this system are not exposed to any shear flow, which allows the reliable tracking of individual cells and AST results to be obtained within 2–3 hours. Furthermore, our system could test the synergistic effect of drugs through two-dimensional gradient generation. Finally, the platform could be directly implemented to new drug discovery and other applications wherein a fast, cost-efficient method for studying the response of microorganisms upon drug administration is desirable.

Schematic of the fabrication process of agar–alginate mixed gel device. (A) The fabrication process of the PDMS mold and the mixed gel. (B) The bonding mechanism of the mixed gel pieces. Upon the addition of Ca2+ ions, alginate is crosslinked and bonds the mixed gel slides together. (C) Cells were cultured on top of the device. (D) Photo of a fabricated mixed-gel device. (E) Schematics of a two-dimensional AST.
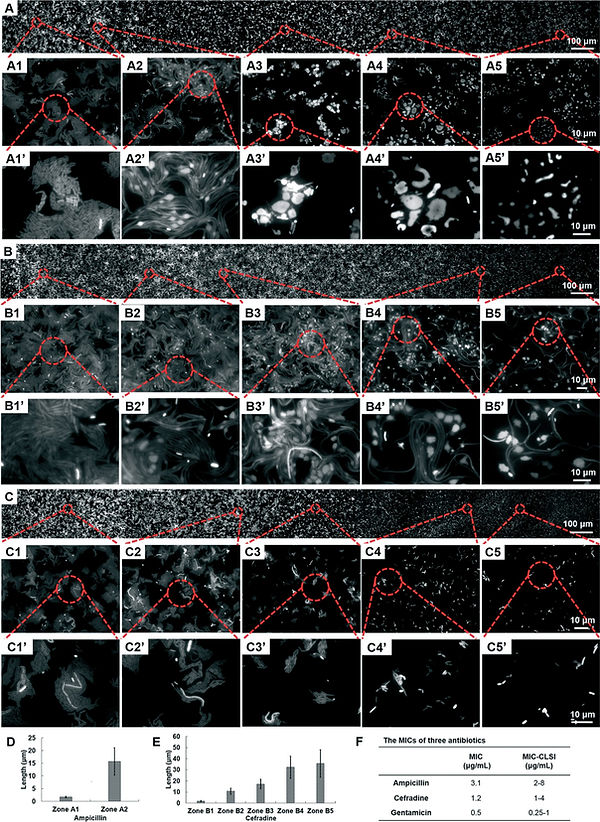
Antibiotic susceptibility testing of GFP E. coli using different antibiotics (A) ampicillin, (B) cefradine and (C) gentamicin. In each fluorescence image, a gradual change from normal bacterial growth to inhibited growth could be observed from left to right. Zoom-ins show the change of cell morphology at different drug concentrations. The bar charts (D–E) show the average length of E. coli cells in each zone examined in the ampicillin and cefradine trials and zones A2 and B2 were chosen as the critical point of inhibition. (F) The table gives the MIC values calculated from image analysis compared with standards from CLSI.
5.A suspending-droplet mode paper-based microfluidic platform for low-cost, rapid, and convenient detection of lead(II) ions in liquid solution (Biosens Bioelectron, 2018)
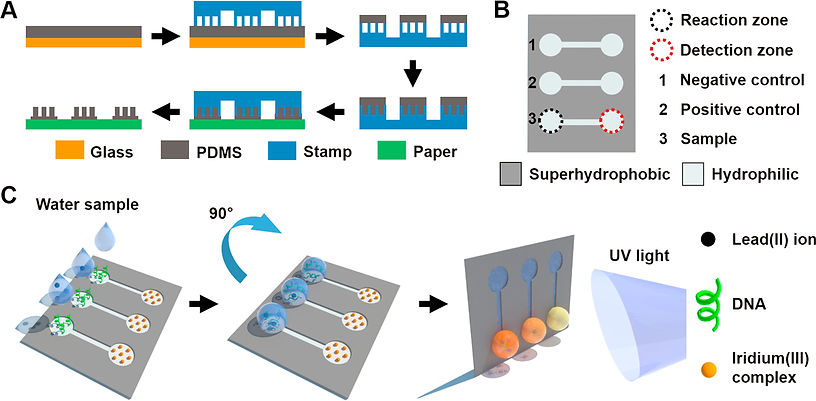
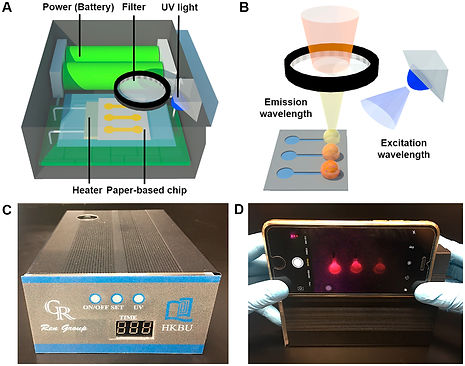
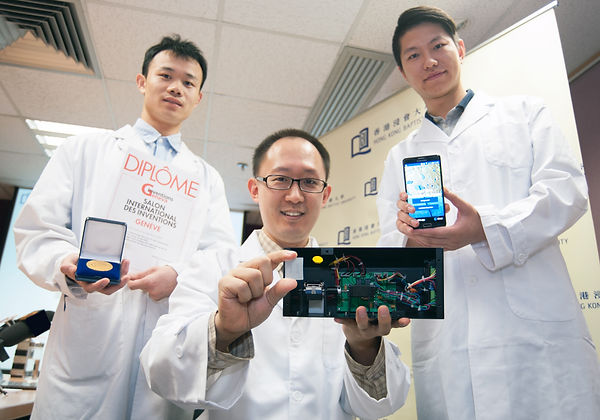
Paper-based microfluidic devices (μPADs) have attracted increasing attention as a new platform for point-of-care (POC) testing and other rapid tests, because of the convenience and low cost in use. However, there is still a major limitation in their functionality, mainly on the capability in handling multi-step reactions. Current μPADs commonly use water-soaking paper to fabricate the channels, so that pump-free liquid driving could be realized utilizing the capillary effect; but such design leaves no room for storage or on-demand manipulation of different liquids on the chip. On the other hand, in many real assays, different reagents need to be held in discrete reservoirs and mixed at specific time points. This major limitation has restricted the applications of μPADs in POC assays.
In this paper we present a new type of μPADs that can address the aforementioned limitation. These devices drive the liquids using surface tension and gravity rather than the capillary force used in conventional μPADs, and can support multiple steps of manipulations on demand. Different from the conventional μPADs that are made in water-soaking paper substrates, the paper substrate we used was art paper, which could prevent the absorption of liquid for a period of time. In such a design, liquid drops are suspended on the surface of the device in designed reservoirs; when the chip is tilted, the liquid drops will move to other reservoirs according to the guidance of channels defined on the surface. To differentiate it from reported μPAD devices that are fabricated with water-permeable paper, we name this new type of paper-based devices suspending-droplet mode paper-based microfluidic devices (SD-μPAD). To fabricate the superhydrophobic barrier pattern on the SD-μPADs, we developed a new method using Teflon micro-templates to produce inexpensive and precisely patterned superhydrophobic coating on paper.
As a demonstration of this new design, we developed a method using the reaction characteristics of iridium(III) complex for rapid, onsite detection of lead(II) ions in liquid samples. Because of the large Stokes shift of the iridium(III) complex probe, inexpensive optical filter systems can be employed, and we were able to make an inexpensive, battery-powered compact device for routine portable detection using a smartphone as a detector, allowing the rapid analysis and interpretation of results on site as well as the automatic dissemination of data to professional institutes, even in poor rural areas of developing countries.